- 📖 Geeky Medics OSCE Book
- ⚡ Geeky Medics Bundles
- ✨ 1300+ OSCE Stations
- ✅ OSCE Checklist PDF Booklet
- 🧠 UKMLA AKT Question Bank
- 💊 PSA Question Bank
- 💉 Clinical Skills App
- 🗂️ Flashcard Collections | OSCE, Medicine, Surgery, Anatomy
- 💬 SCA Cases for MRCGP
To be the first to know about our latest videos subscribe to our YouTube channel 🙌
Introduction
The body regulates blood pH to stay within a narrow range of 7.35 to 7.45. Fluctuations outside of this range can be detrimental to cellular processes. Enzymatic function can be hindered at suboptimal pH ranges, leading to cellular death.
To keep blood pH within the optimal range, multiple homeostatic mechanisms regulate processes and molecules which contribute to pH:
- Chemical acid-base buffer systems
- Respiration, and its control by the respiratory centre
- The kidneys
Chemical acid-base buffer systems can react to changes in pH within seconds to minutes, and the respiratory centre can also react within minutes. The kidneys, while being the most powerful of the regulatory processes, take hours to days to respond.
This article will cover the different homeostatic mechanisms which regulate blood pH.
pH
pH is a scale that describes how acidic or basic a fluid is.
The pH scale is logarithmic and is inversely proportional to the concentration of hydrogen ions (H+) in the fluid. This means that a difference of one pH unit is equivalent to a tenfold difference in hydrogen ion concentration.
When the acidity of a solution is increased by adding H+, the pH value decreases. The pH scale runs from 0 to 14, with a pH of 7 indicating neutrality, where the concentration of hydrogen ions equals the concentration of hydroxide ions (OH–).
Blood pH buffer systems
Buffer systems work by neutralising added acid or base to resist changes to pH. For example, when H+ is added, the buffer system acts to ‘mop up’ excess H+. When H+ is low, or excess base is added, the buffer can ‘donate’ its own H+ to the solution to try and minimise the pH change.
Bicarbonate buffer system
The bicarbonate buffer system is quantitatively the largest buffer system of the blood. Carbonic anhydrase is a metalloenzyme found in the blood that catalyses the reaction of carbon dioxide (CO2) and water (H2O) to form carbonic acid (H2CO3), as well as the inverse reaction.
Carbonic acid is a weak acid and can donate a hydrogen ion to form bicarbonate (HCO3–), which functions as a weak base. These reversible reactions function as a buffer system. Bicarbonate ions bind with the H+ from introduced acids, while introduced bases receive a donated H+ from carbonic acid, effectively neutralising them.
Le Chatelier’s principle
Le Chatelier’s principle, also called the equilibrium law, explains that when a system in an equilibrium state changes conditions, it will change its composition in such a way as to reduce the effect of the change. This can be used to explain the basic movements of the bicarbonate buffer system.
CO2 + H2O <-> H2CO3 <-> HCO3– + H+
The bicarbonate buffer is in an equilibrium state within the body, under normal physiological conditions. If one substrate is increased, such as CO2, the equilibrium will shift to the right, and generate more H2CO3 as well as HCO3– + H+, to offset the change in CO2 concentration. If an increase in H+ occurs, the equilibrium will shift to the left, and more CO2 and H2O will be generated, decreasing the H+ concentration. The same would occur if CO2 were to be removed.1
The buffer system works to keep the pH within the optimum range unless the amount of acidic or basic additions overcomes the reserve amount of buffer molecules in the extracellular fluid.
Other buffer systems
Two other intravascular buffers aid in pH homeostasis. The first is proteins, of which haemoglobin is the most numerous and quantitatively the strongest. Histidine residues on the haemoglobin function as proton (H+) acceptors at physiological pH levels, noting that the buffering capacity of haemoglobin increases in its deoxygenated state.
The other buffer is the phosphate buffer system. Inorganic phosphate (HPO42–) can reversibly bind free hydrogen ions. Compared to bicarbonate its concentration in the blood is low, and therefore its impact on blood pH is low. However, it is critical for buffering the pH of urine.
Respiratory regulation of acid-base balance
CO2 is generated from cellular metabolism. It enters the bloodstream and is incorporated into the bicarbonate buffer system or dissolved in the plasma.
Respiration affects blood pH by dictating the rate of removal of CO2 from the blood.
Hypoventilation causes CO2 retention, and the bicarbonate buffer system compensates by forming carbonic acid, lowering blood pH. Hyperventilation, on the other hand, increases CO2 removal and thus pushes the bicarbonate buffer system towards forming CO2 and H2O while decreasing H+, thereby increasing blood pH.
Ventilation can also be used by the body to alter the pH in response to changes caused by other means. An increase in blood H+ concentration signals the respiratory centre via chemoreceptors to stimulate alveolar ventilation, increasing the rate and/or depth of respiration. By removing CO2 via respiration, the body can increase blood pH.
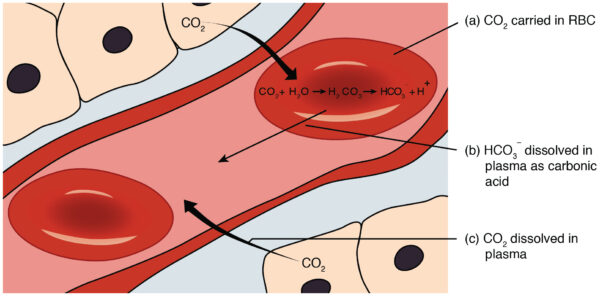
Renal regulation of acid-base balance
The kidneys control acid-base balance by removing excess acid or base in the urine, and reabsorption and generation of HCO3–.
Substantial amounts of HCO3– are continuously filtered into the tubules. However, most is reabsorbed to conserve the buffer system of the extracellular fluid. H+ is also secreted into the tubular lumen, which includes the non-volatile acids generated by metabolism.
HCO3– must react with H+ to form H2CO3 within the lumen before it can be reabsorbed. Thus, the reabsorption of HCO3– is linked to the secretion of H+ into the tubular lumen.
Only a small amount of H+ can be excreted in the urine in its ionic form. Two main buffers in tubular fluid aid H+ excretion: the phosphate buffer and the ammonia buffer.
Phosphate buffer
The phosphate buffer can aid tubular cells to replenish extracellular HCO3– when stores are low.
Monohydrogen phosphate (HPO42-) is concentrated in tubular fluid due to a low reabsorption rate. Most H+ excreted into tubular fluid combines with HCO3–. However, once all HCO3– has been reabsorbed, the H+ can be combined with HPO42- and excreted as a salt (NaH2PO4). The removal of H+ allows the bicarbonate buffer system to create more HCO3– as per Le Chatelier’s principle, which can then be reabsorbed.
Ammonia buffer
The ammonia buffer works in two areas to ‘trap’ H+ in the urine and increase the HCO3– of the body. In the collecting tubules, H+ is generated from CO2 and H2O in the tubular cells. The H+ is actively secreted into the lumen, where it combines with ammonia (NH3) to form an ammonium ion (NH4+). The collecting ducts are almost impermeable to NH4+ compared to NH3, so H+ is ‘trapped’ in the lumen and excreted. For every H+ generated, one HCO3– molecule is reabsorbed into the bloodstream.
In the epithelial cells of the proximal and distal tubules, as well as the thick ascending loop of Henle, metabolism of glutamine molecules generates two HCO3– molecules and two NH4+ molecules. While the NH4+ is excreted into the lumen, the HCO3– is transported across the basolateral membrane and taken up by peritubular capillaries.2
When there is alkalosis of the extracellular fluid, the kidneys secrete less H+ into the urine, and fail to reabsorb all the filtered HCO3–. Due to the buffering nature of HCO3– in the bicarbonate buffer system, this loss of HCO3– raises the extracellular H+ concentration and thus acts to correct the alkalosis.
When there is acidosis of the extracellular fluid, the kidneys secrete additional H+ which also leads to reabsorption of higher amounts of filtered HCO3–. New HCO3– is also produced by the buffers in the tubules. The new and reabsorbed HCO3– act via the bicarbonate buffer system to correct the acidosis.
Clinical relevance – respiratory acidosis and metabolic compensation
Respiratory acidosis is defined as CO2 accumulation (hypercapnia) due to a decreased respiratory rate and/or respiratory volume (hypoventilation). Disorders which cause hypoventilation include:
- Neuromuscular disorders, and those that cause muscle weakness: myasthenia gravis, muscular dystrophy, amyotrophic lateral sclerosis (ALS)
- Obstructive, restrictive, and parenchymal pulmonary disorders: COPD, asthma, sleep apnoea, airway oedema
- Respiratory drive disorders: brainstem stroke, CNS depressant medications such as ethanol, benzodiazepines, opioids
- Chest wall deformities
Respiratory acidosis may be acute or chronic. This can be determined by the amount of metabolic compensation. The kidneys typically respond to respiratory acidosis by increasing hydrogen ion secretion and bicarbonate reabsorption.
However, the kidneys take hours to days to respond to changes in acid-base status. Therefore, any respiratory acidosis accompanied by increased bicarbonate ions may be a chronic disorder, as the kidneys have had sufficient time to respond to acidaemia.
To learn more about the investigation of acid-base disturbances, see our guide to arterial blood gas interpretation.
Key points
- Blood pH is regulated by multiple homeostatic mechanisms, including chemical buffers, respiration, and the kidneys.
- The bicarbonate buffer system can act within seconds to minutes to counteract changes in pH, while the lungs take minutes, and the kidneys take hours to days.
- Respiration alters acid-base balance by changing the carbon dioxide concentration of the blood, while the kidneys work by changing bicarbonate generation and reabsorption, as well as modifying hydrogen ion excretion.
- The phosphate buffer and the ammonic buffer in the kidneys work to ‘trap’ hydrogen ions in the urine, while also generating bicarbonate ions.
Editor
Dr Chris Jefferies
References
- Stephen Lower. Le Chatelier’s Principle. Published in 2022. Available from: [LINK]
- Hall JE, Hall ME. Guyton, and Hall Textbook of Medical Physiology. 14th edition. Published in 2021.
Image references
- Figure 1. OpenStax College. 2325 Carbon dioxide transport. Licence [CC BY 3.0]